New research from Rothamsted
Novel tool will give breeders more predictive accuracy for seed development and growth.
Scientists at Rothamsted have developed a novel mathematical model for seed germination. The new tool is derived from field data and marks a significant improvement in accuracy over previous models.
Germination models use water and temperature as the main drivers of seed activity. Currently these are based largely on water potential, which is the energy that drives water to move from one system to another. This is challenging to measure accurately in the field. The new model uses water content which is much easier to measure.
These models are essential to the seed industry as it is important to develop lines where each individual seed germinates at roughly the same time and grows uniformly. This is particularly important for crops like carrots or onions where having similar sized plants makes them more profitable.
Seed suppliers must therefore test seed samples to ensure a certain germination rate is met, a process that is difficult and time consuming. So modelling is used to assess each batch based on a given sample.
However, current models do not always accurately reflect field conditions
That is where the new model comes in. Water content is much easier to measure accurately in situ than the drivers of water potential. That makes the new model far more likely to accurately predict germination times in field situations.
“This is the first time a germination model has been able to be developed using field data,” said Dr Xiaoxian Zhang, corresponding author of the new study. “Seed germination is a crucial stage in plant development, intricately regulated by various environmental stimuli. Understanding these interactions is essential for optimizing planting and seedling management but remains challenging due to the trade-off effects of environmental factors on the germinating process.”
The research team created a new model by viewing seed germination as a dynamic process. To validate the model, they conducted field experiments by drilling wheat seeds at different dates to establish a temperature gradient and in different plots to create a soil water content gradient.
Comparisons between the experimental data over a period of seven years and calculated results show that the model accurately reproduces all germination patterns and the subsequent seedling tillering with a 95% fit.
“In germination there a trade-off effect of soil water on bioavailable water and oxygen. Introducing a physiological dimension enables seed germination and the subsequent tillering process to be modelled as a continuous physiological process, providing deeper insight into plant growth dynamics,” said Zhang. “We believe this new approach offers a genuinely new approach to germination modelling and for the first time takes on tiller number. We also hope that it will be more stable than the hydrothermal time models and hence more useful for field application,” he added.
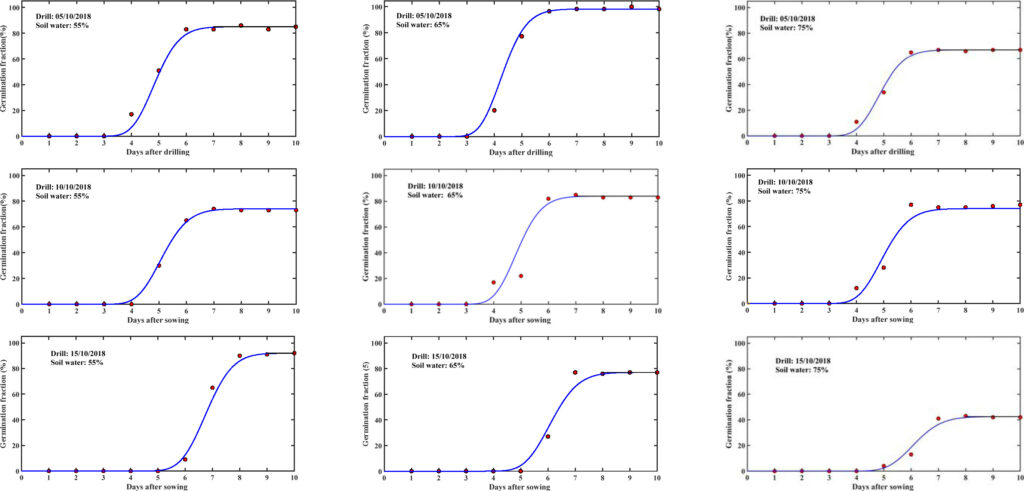
Read the paper: A new conceptual model for seed germination and seedling tillering of winter wheat in the field
Abstract
Seed germination is a crucial stage in plant development, intricately regulated by various environmental stimuli. Understanding these interactions is essential for optimizing planting and seedling management but remains challenging due to the trade-off effects of environmental factors on the germination process. We proposed a new conceptual model by viewing seed germination as a dynamic process in a physiological dimension, with the influence of environmental factors and seed heterogeneity characterized by a germination speed and a dispersion coefficient. To validate the model, we conducted field experiments by drilling wheat seeds at different dates to establish a temperature gradient and in different plots to create a soil water content gradient. Comparisons with our experimental data and literature results show the model accurately reproduces all germination patterns and the subsequent seedling tillering, with R2 > 0.95. Our results reveal that within suboptimal temperature range, the seed germination increases asymptotically with temperature, and that as soil water content increases, the germination speed increases initially before decreasing, illustrating the trade-off effect of soil water on bioavailability of water and oxygen. Introducing a physiological dimension enables seed germination and the subsequent tillering process to be modelled as a continuous physiological process, providing deeper insight into plant growth dynamics.
1. Introduction
Seed germination is critical not only to plant survival but also to post-germination plant development such as fitness, timing of flowering and dynamics of the plant community. Difference between seeds in their response to environmental stimuli is an evolutionary strategy to improve plant survival in natural systems. In managed ecosystems, however, it is desirable for seeds to germinate simultaneously, and germination uniformity is thus a seed quality trait.
Seed germination is a complex physiological process modulated by environmental cues including light, oxygen (O2), temperature and the amount of water available for seeds to imbibe. The onset is water uptake of the quiescent dry seed and the end is the emergence of the embryonic axis out of the seed coat. Several key stages, including solute leakage during imbibition, initiation of metabolic activity for protein synthesis and emergence of the radicles, are distinguishable in germination, many of which can be visualized non-invasively through imaging technologies. Seed germination has received less attention compared to other topics in plant science, but the advances in molecular technologies over the past few decades have substantially improved our understanding of the genes and interlocked hormone networks that regulate seed germination. The response of gene expression to environmental cues in seed germination has been well documented, and network modelling of the transcriptional interactions involved in seed germination has also been constructed to link specific genes and pathways to seed germination and dormancy. Quantitative modelling of seed germination is largely based on the hydrothermal time concept assuming that temperature and water are the main environmental stimuli of seed germination. A key feature of the hydrothermal time models is that when temperature and water potential exceed their respective base values (below which seeds remain metabolically inactive), the seed needs to accumulate a sufficient hydrothermal time for its radicle to break the seed coat. Seed heterogeneity is described by viewing the base temperature and/or water potential as random variables. The hydrothermal time model was initially developed for seed germination between the base and the optimal temperatures, and has since been generalized to cover a wide range of temperatures as experiments revealed that average germination does not increase linearly with temperature after temperature exceeds a threshold. The improved hydrothermal time models include the halo-time model to account for salinity and the oxygen-time model to represent O2 stress.
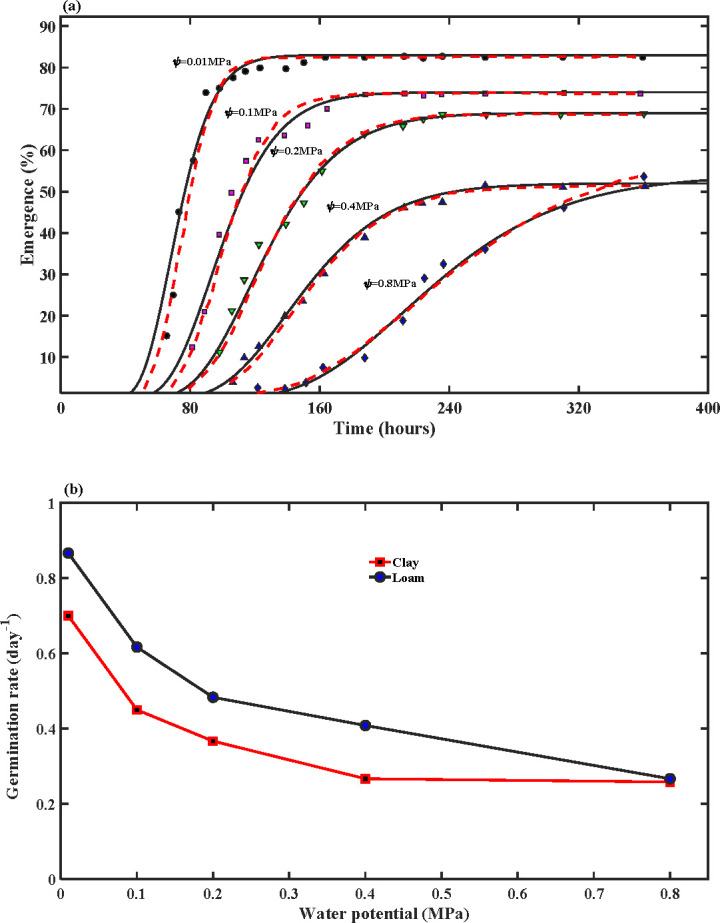
Seeds need to absorb sufficient water and thermal energy to germinate. Physically, the linear dependence of hydrothermal time on temperature and water potential, as assumed in the hydrothermal time models, means that the rates at which water and heat flow from soil into the seed are proportional to a water potential gradient and a temperature gradient respectively. While this is true for seed germination in polyethylene glycol on Petri dishes, where the seed–water contact area is constant and the only resistance to seed imbibition is the ability of seed coat to adsorb water, its application to the field could be problematic. Seed imbibition of water from the soil occurs through the seed–water interface, and the imbibing rate depends not only on water potential difference between seed and the water–seed interface, but also on the ability of soil to transport distant water toward the seed and the water–seed interfacial areas to imbibe water, both varying nonlinearly with water potential. Apart from this, temperature fluctuates in the field and the atmospheric O2 needs to overcome various resistances before becoming bioavailable to seeds. Hydrothermal models use an oxygen time to describe O2 sensitivity, but bioavailable O2 in the field is not an independent environmental cue; it is controlled by soil water and pore-scale soil structure, thereby varying with soil texture and soil water content.
This paper aims to propose a new conceptual model to simulate seed germination in the field. We view the metabolic reactions associated with seed germination as a dynamic process in a physiological dimension. The fluctuations in environmental stimuli and seed heterogeneity result in uncertainties in the metabolic reactions, in that physiological advancement that a seed makes at different times and physiological stages varies. We show that such variations can be modelled by a partial differential equation, with the mean of the variations represented by an average germination speed and the variance by a dispersion coefficient. To test the model, we conducted field experiments to measure the germination and seedling development of winter wheat seeds drilled at different dates (to generate a temperature gradient) in plots with different soil water contents (to generate a soil moisture gradient). The novelty of introducing a physiological dimension is that it allows seed germination and subsequent seedling development to be modelled as a continuous physiological process.
The challenge for hydrothermal time models, which require water potential, is that, due to technological limitations, measuring soil water potential higher than 200 kPa in the field using the best available methods is difficult. This value is much lower than the optimal soil water potential for wheat seeds. Additionally, in situ measurement of dissolved O2 is also challenging. This is why most hydrothermal time models were derived from Petri-dish experiments and have limited applications in the field. This paper aims to bridge this gap.
You can read the full paper here: https://royalsocietypublishing.org/doi/10.1098/rsos.240723
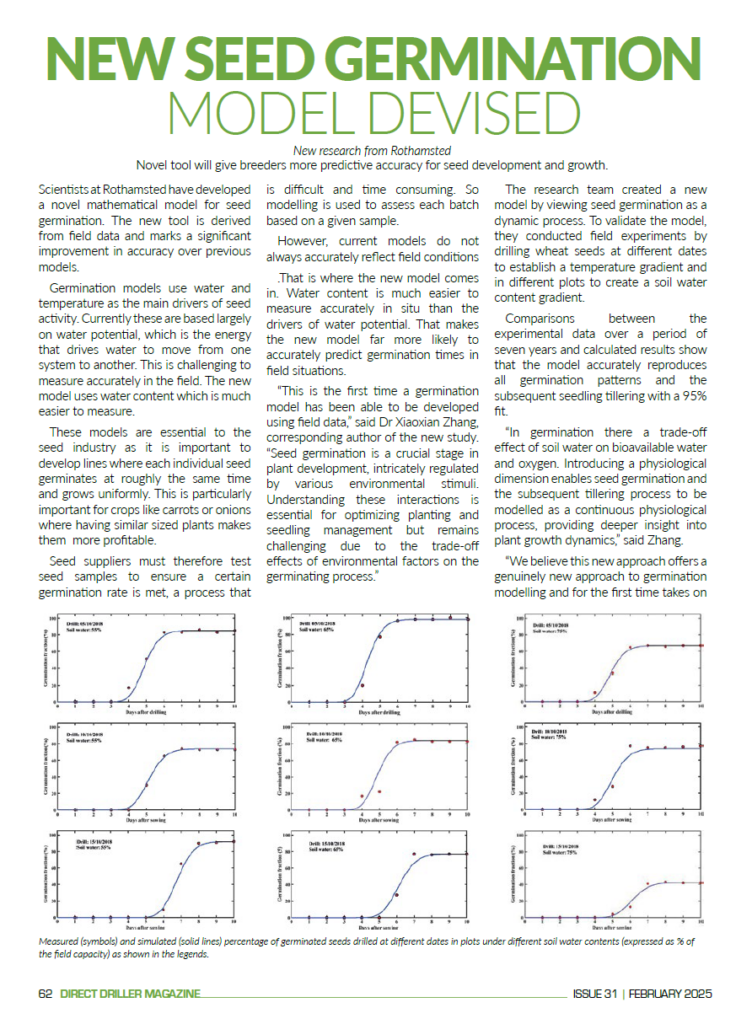
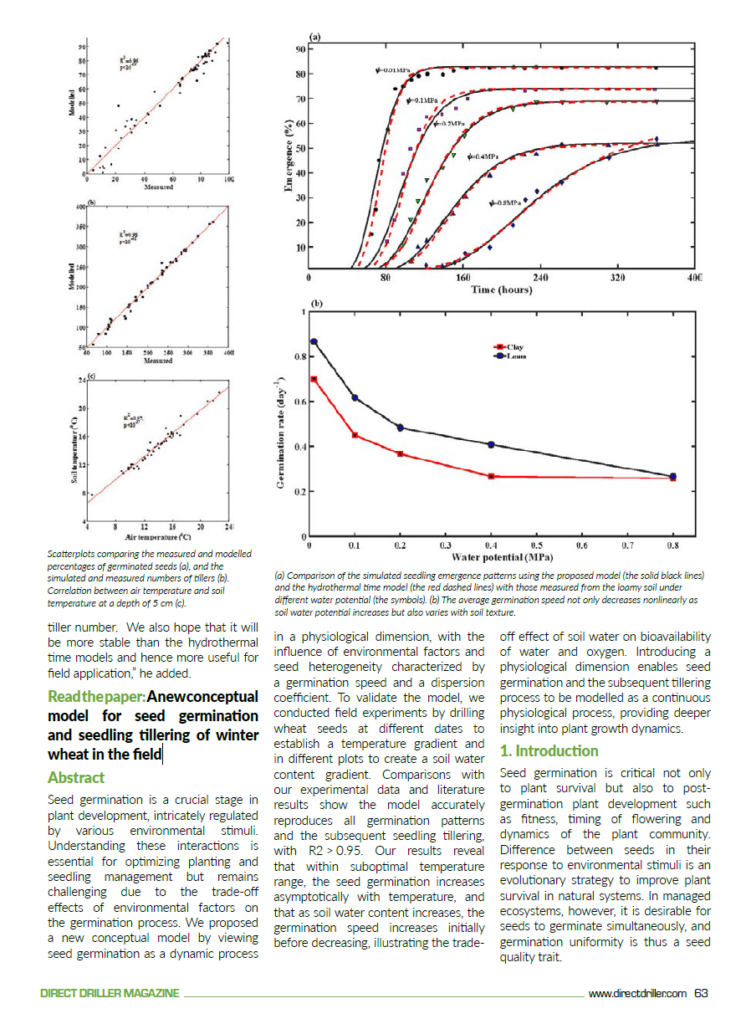
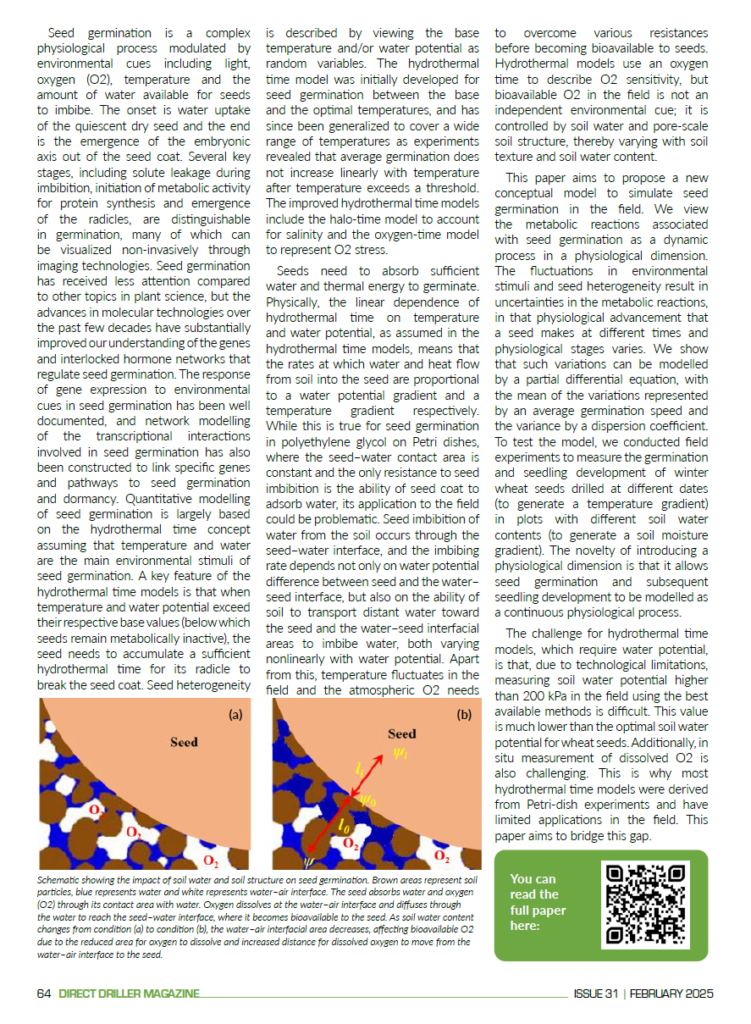