Billions are being invested in gene editing globally, but what innovations will that investment deliver? Tech Farmer visited the World AgriTech Innovation Summit to gain a global perspective on what it might deliver for UK farmers.
Written by Mike Abram
There’s been no shortage of global investment in gene editing, even if venture capital funding in the area has plateaued in recent years.
Since the first research paper demonstrating genome editing of plant genomes using CRISPR/Cas9 was published in 2013, somewhere between $4bn and $6bn has been ploughed into start-ups applying the technology in agriculture, while similar, if not higher amounts have been invested by seed companies of all sizes, according to Syngenta head of seeds research Gusui Wu.
“Significant investment or funding has also happened in the public sector in plant gene-editing research,” he told delegates at the World AgriTech Innovation Summit in San Francisco.
Progress has been made in turning that investment into commercialised products. The US Department of Agriculture (USDA), for example, has granted exemptions for (effectively approving) 53 gene-edited traits in 17 crops, as of January 2024.
“At the same time, we have not seen the kind of big impact, large scale commercialisation of gene-edited products in the market yet,” Gusui acknowledged.
That’s not totally surprising, he argued. It took over 10 years from the initial publication of research for the last disruptive technology in plant breeding, transgenic genetically modified traits, until the first blockbuster commercial trait in Roundup Ready soybeans.
And it wasn’t simply because of the regulatory process, although that played a part. It was as much that associated application technologies, such as methods to introducing traits into elite varieties, also needed to be developed alongside the plant transformation technique.
The same is true for gene editing, where enabling technologies are also required. “The good news is that in the past five years significant progress has been made,” Gusui said. “And we’re now approaching a turning point for gene-editing new breeding technology.”
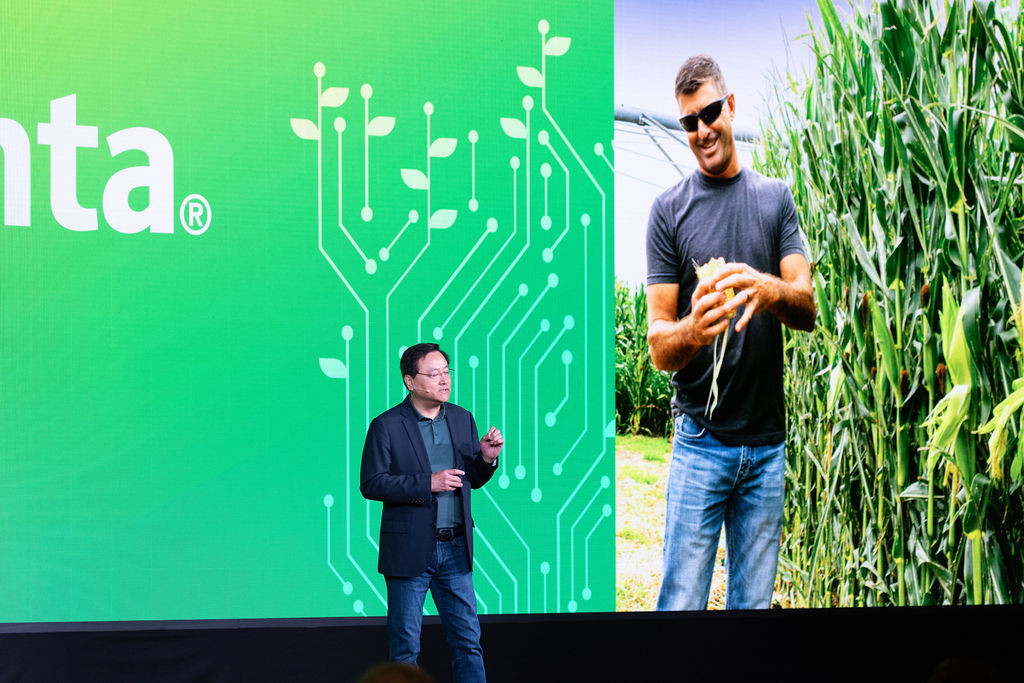
Progress includes development of alternative CRISPR/Cas systems by companies like Inscripta, Pairwise and Syngenta to the CRISPR/Cas9 system originally used, which overcomes some of the Cas9 limitations, including its murky intellectual property path, Gusui suggested.
Syngenta has also developed a method of introgressing any trait into elite lines in just one generation, rather than six or seven generations it usually takes. “These types of technologies are very important for the success of gene editing in agriculture,” he said.
“Perhaps the largest opportunity is how we apply gene editing to complex traits,” he noted. “For those traits it is difficult to make simple edits. Instead, we will likely need multiple gene modifications and that is a step change that can be brought by CRISPR.”
Making multiple gene edits at the same time, including different types of edit such as turning genes on or off, or regulating gene expression up or down, will be crucial to making transformational changes, rather than just incremental improvements, according to Inari CEO Ponsi Trivisvavet.
“We are targeting 10-20% yield improvements in three key crops – corn, soybeans and wheat – without adding any resources,” Ponsi said. “In corn, we’re also targeting a 40% reduction in water use and 40% reduction in nitrogen by improving resource use efficiency.”
Inari is using two technology platforms to help achieve those goals. The first, artificial intelligence-powered predictive design help scientists understand what genes to target and express more effectively, she explained.
“For the second part, we use multiplex gene editing to not only turn genes on and off but also think about increasing or decreasing gene expression, like dimming a light switch or making it brighter.
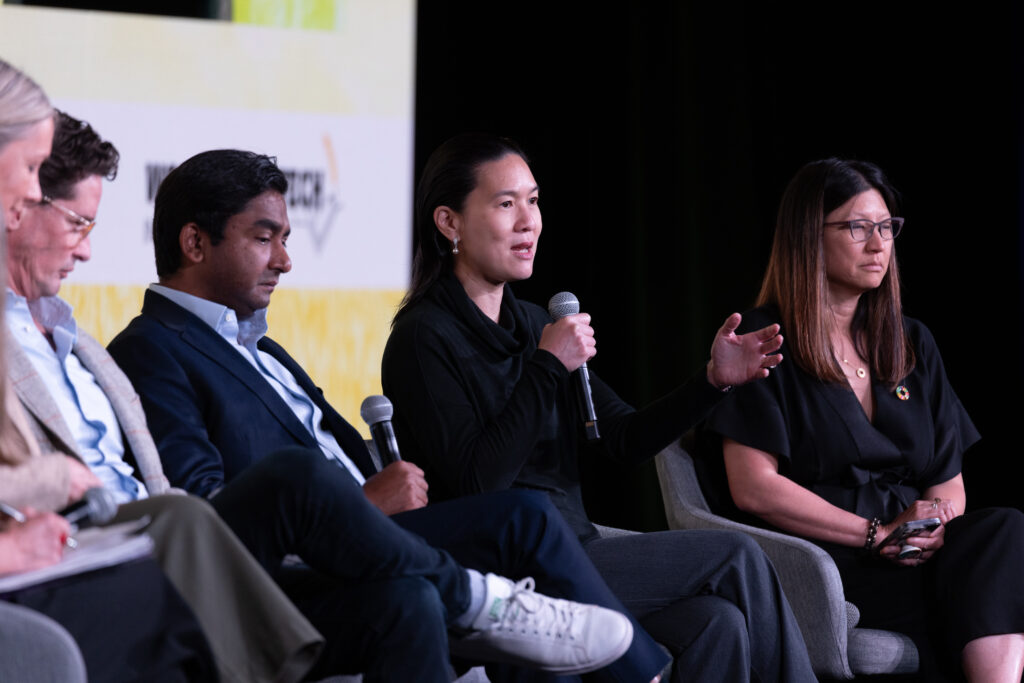
“And we only use tools that work on natural genes, meaning when we edit wheat, for example, we only edit wheat genes.”
That approach has led to the development of a potentially higher yielding gene-edited wheat. “There is no simple answer for how to increase yield. Through predictive design we are working to build an understanding of the wheat genome’s complexity and create a blueprint for updating the plant’s architecture with our multiplex gene editing,” Ponsi said.
“In this project we’ve explored edits to address grain size, quantity and weight, as well as the number of tillers.”
The first trials of the gene-edited wheat will be in Australia, after Inari signed a collaboration with Australian breeding company InterGrain. “We delivered our gene-edited seeds into Australia, where they are currently in a quarantine greenhouse. Once released they will be further evaluated in local field conditions in field testing.”
Inari’s technology is transferable to other geographies and crops, she added.
Another example of a gene-edited wheat in development was given by Prof Eduardo Blumwald, professor of cell biology at University of California, Davis. His research is looking to address the challenge of reducing synthetic fertiliser use in crops, such as wheat, while maintaining production.
The starting point for his research was examining plant exudates from roots. “The plant communicates with the environment through chemicals,” he explained. “They extrude chemicals all the time through their roots, and with that they can talk with the environment.”
The reason why that is important is that in the soil are nitrogen-fixing bacteria – 80% of our air is nitrogen and only those bacteria can fix that nitrogen into ammonia which plants can use.
“The problem is only 10-15% of the bacteria in the soil can do that. But they don’t because in agriculture soils need oxygen, and oxygen inhibits the nitrogenase enzyme that can fix nitrogen.
“So we started analysing the plant exudates to see which one of those chemicals can induce the production of biofilms in soil. Why a biofilm? Because the biofilm is practically impermeable to oxygen.
“So although there is oxygen in the soil, bacteria surrounded by the biofilm start behaving like hippies by forming colonies and sharing resources,” he said.
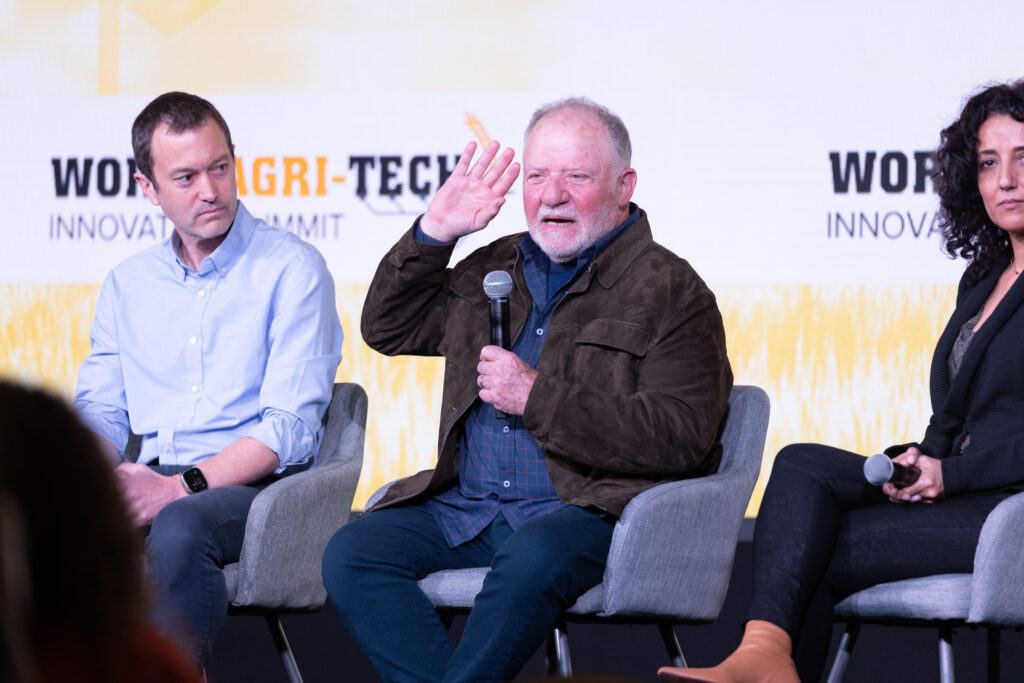
While surrounded in the colony by biofilm the bacteria fix nitrogen and produce ammonium.
“They’re happy, the plants are happy, and the farmers are happy as they can reduce the amount of nitrogen applied.”
Finding exudates that induced biofilm production was not easy, Eduardo stressed. “We screened almost 3000 chemicals and only found a few candidates.”
The next step was to understand the complex metabolic pathway that produced the chemical in the plant. Instead of over expressing the pathway for the plant to produce more, Eduardo silenced the enzyme that uses the chemical in the plant, so it accumulates, and more is ultimately extruded.
“It sounds easy, but it’s not,” Eduardo said. “But we did it in rice and in wheat.”
The result are crops that need 50% less fertiliser to grow, albeit ones that currently come with a 25% loss in yield in wheat. That could be improved, Eduardo hopes, with further development in the hands of a major plant breeder.
“We think there could be some issues with the way the plants are assimilating the nitrogen, so we are working with the breeder to find better varieties. We believe that in very old cultivars there might be some great candidates.”
Biotechnology firm Switch Bioworks is also investigating the use of microbes to fix nitrogen to replace synthetic nitrogen fertiliser. Its initial research focused on corn, Elizabeth Ordeman, senior research associate at the firm, told Tech Farmer.
“We are isolating the best colonisers and nitrogen fixers that already live around corn roots, and then engineering them to share nitrogen with the plant.”
Typically, wild-type bacteria won’t share nitrogen because of the high energy intensity required for nitrogen fixation. “The resource is very precious for bacteria. What we do is add a genetic switch to microbes already great at fixing nitrogen that allows them to release ammonia that can be used by the plant.”
While the concept of engineering a microbial biofertiliser product isn’t unique to Switch Bioworks, the key differentiator for the company is the separation of the process of building the microbial population and producing fertiliser.
“If you engineer a microbe that always produces fertiliser, since it’s an energy intensive process, the microbe can never grow or compete well in the soil, and you don’t end up with that many bacteria producing fertiliser in the field,” Elizabeth explained.

Switch Bioworks engineered bacteria incorporates a sensor that leads to the activation of ammonium release later in the season, once the population has built. “You get this period where the microbes grow and colonise the root, and then when they are at a high number and the plant needs nitrogen the most, you turn on the ammonium production.”
How exactly that happens is the firm’s intellectual property, but Elizabeth said there were a number of different options available that could serve as the trigger, such as changes in the soil, changes in the bacteria or something related to plant exudates to name just three.
But building the microbial population first was critical, she stressed.
“Every time the population doubles, it doubles the amount of fertiliser the bacteria can produce. It’s how we think one ounce of dried microbes will be able to inoculate an entire acre and replace up to a 100 lbs/acre (112 kg/ha) of nitrogen.”
US start-up Napigen is specialising in gene editing of mitochondrial DNA, using it to develop male sterile wheat plants that can be used in hybrid breeding of wheat.
Development of hybrid wheat has been held back commercially because of the difficulty in producing a viable hybrid seed production system in the crop, with one key challenge the development of male sterile parents, Dr Hajime Sakai told Tech Farmer.
“Currently there is no efficient, stable method of producing male sterile plants, but we hope our technology will enable that.”
Male sterility in rice, vegetables and wheat occurs naturally through genetic differences in the mitochondria, so Napigen is mimicking that through its gene-editing process.
The result, according to Hajime, will be a reliable male sterility trait in elite wheat lines in a much cleaner genetic background than anything currently available.
Once proven, he expects to partner with seed companies to develop hybrid wheat that could increase wheat yields by anything from 15% to even doubling current yields.
Napigen is also using its technology to develop other products, such as herbicide-resistant crops that will not cross-pollinate with weedy plants.
Gene-edited nematode resistant potatoes being developed
Israeli biotech start-up GeneNeer is developing gene-edited potato traits using its proprietary “Superlines” platform, including nematode and disease resistance.
Gene editing in potatoes has proven challenging, even with the newly developed gene-editing tools, CEO and co-founder Dr Kinneret Shefer says, partly because of the inability to completely remove the gene-editing machinery.
As long as foreign DNA is no longer present in the final gene-edited product, most regulatory bodies across the world do not regard the edited crop to fall under genetically modified regulations.
However, removing the DNA is difficult in potatoes due to vegetative clonal propagation from tubers rather than from true seeds. The complexity of the potato genome, particularly its tetraploidy – four copies of each gene – is another complicating factor, which makes generating new traits challenging.
GeneNeer is overcoming these challenges through its Superlines platform, which simplifies the gene-editing process by creating tissue cultures of leading varieties that serve as innovation platforms for researchers to modify genes and create improved traits.
This eliminates the need for crossing or single cell assays to remove the gene-editing machinery as well as reducing the time to produce improved traits.
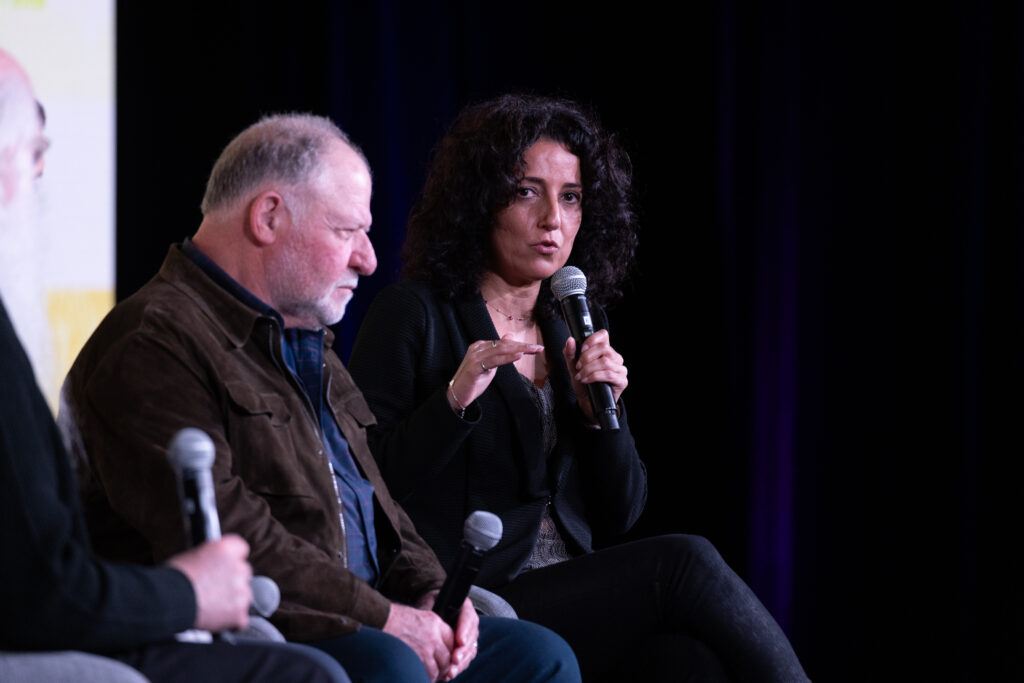
One of the first traits arising from GeneNeer’s platform is a gene-edited potato with nematode resistance.
“We can use our technology to silence genes in a tissue-specific manner,” Kinneret explains. “Our manipulation occurs in the root tissues, where we silence some specific genes which prevent the nematode from proliferating. Even if a nematode attacks the potato, it cannot complete its lifecycle, cannot lay eggs and use the potato as a host.”
Initial work is being done in three varieties – Russet, Atlantic and Desirée – against both potato cyst and root knot nematodes.
The second development is aiming to solve what Kinneret suggests is a growing problem in potato production in North America – verticillium wilt.
“The reason why it is a growing problem is the push in Canada for faster maturation of the potato crop is genetically linked to greater susceptibility to the fungus causing wilt, Verticillium dahliae.
“It is very hard to target with chemicals, and because of the genetic link, it is difficult to solve with traditional breeding. However, with gene editing we can break that link,” she says.
What is gene editing?
Gene editing, also known as genome editing, at its most basic changes a DNA sequence in a living cell. It’s only been practically possible since 2012, when Nobel laureates Jennifer Doudma and Emmanelle Charpentier discovered the CRISPR-Cas9 method for precisely editing genomes.
Using such a tool allows breeders and plant scientists to “cut” a specific DNA sequence and rely of cells’ natural DNA repair mechanisms to introduce changes at that site. Think of it like a word processor used to edit documents – adding, deleting and replacing letters in the cell’s natural genetic code.
Editing just a single gene can have important effects. Most gene editing to date in food and agriculture are the result of simple edits like turning off, of “knocking out” genes. Examples include improved soybean oil with reduced saturated fatty acids, GABA-enriched tomatoes with higher levels of amino acids to lower blood pressure and browning-resistant mushrooms to prolong shelf life.
But simple edits may not be sufficient to tackle some challenges. The size and complicated nature of many plant genomes increases complexity, while some functions, such as water use efficiency or increased yield, can be driven by multiple genes working together rather than a single native gene.
Enhancements might also require different types of edit, where one gene might need to be knocked out, while other genes’ expressions need to be amplified or suppressed.
That’s where multiplex gene editing can help. It involves making a variety of edits and edit types to multiple genes at the same time. Coupled with the latest advances in genomics and artificial intelligence it provides the opportunity to address biological complexity resulting in accelerated crop improvements.
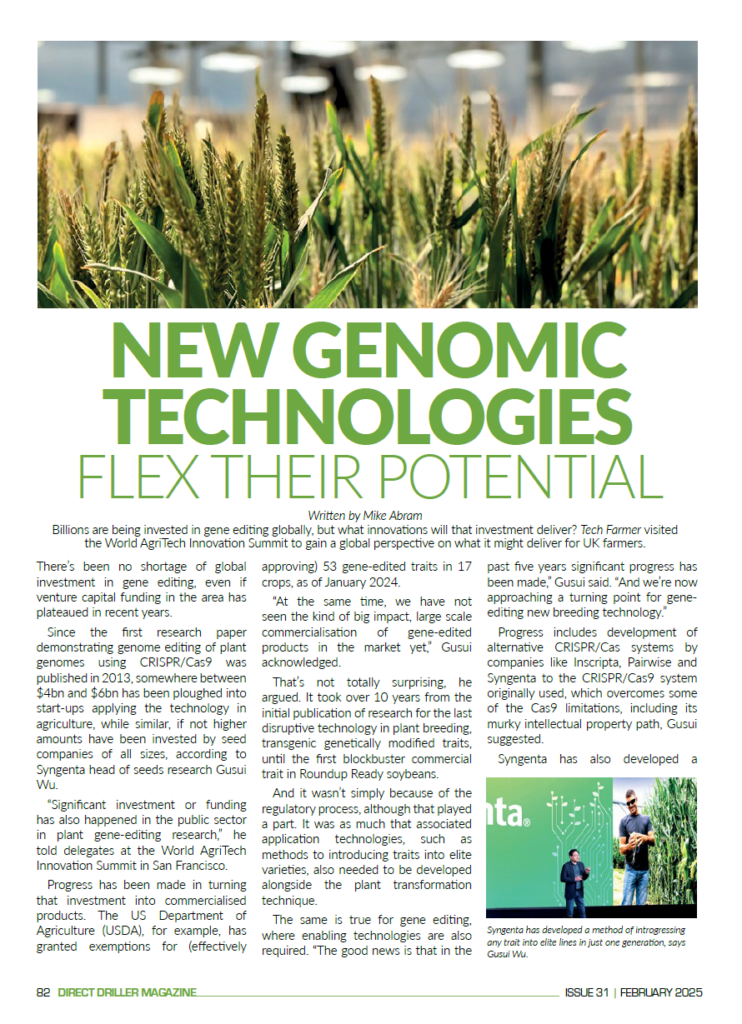
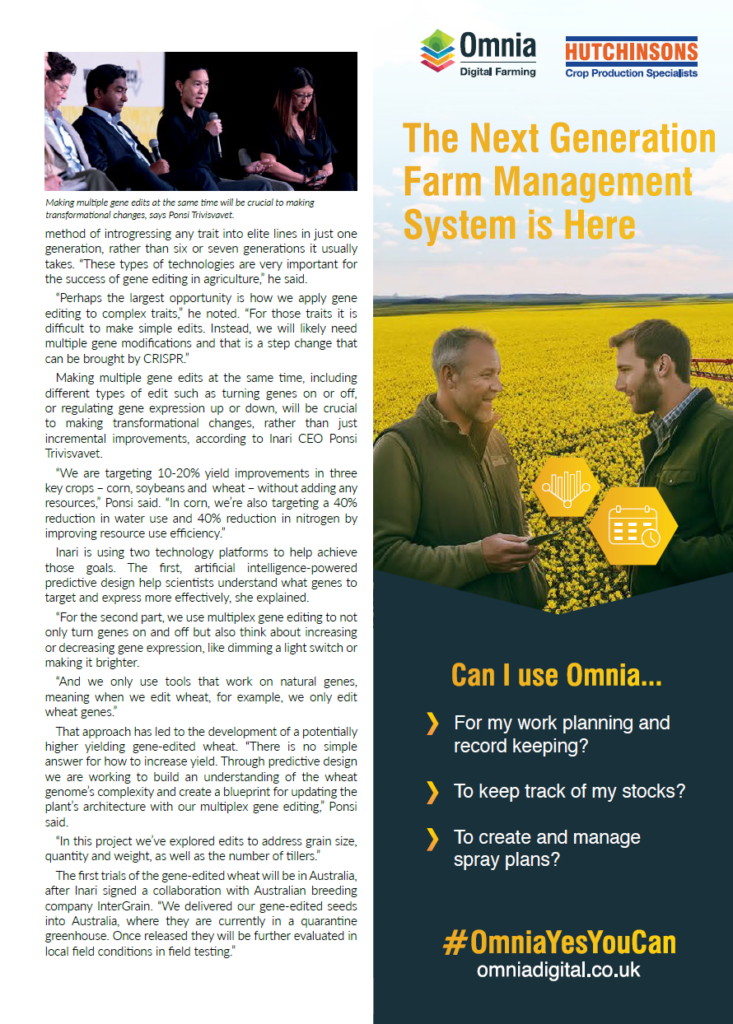
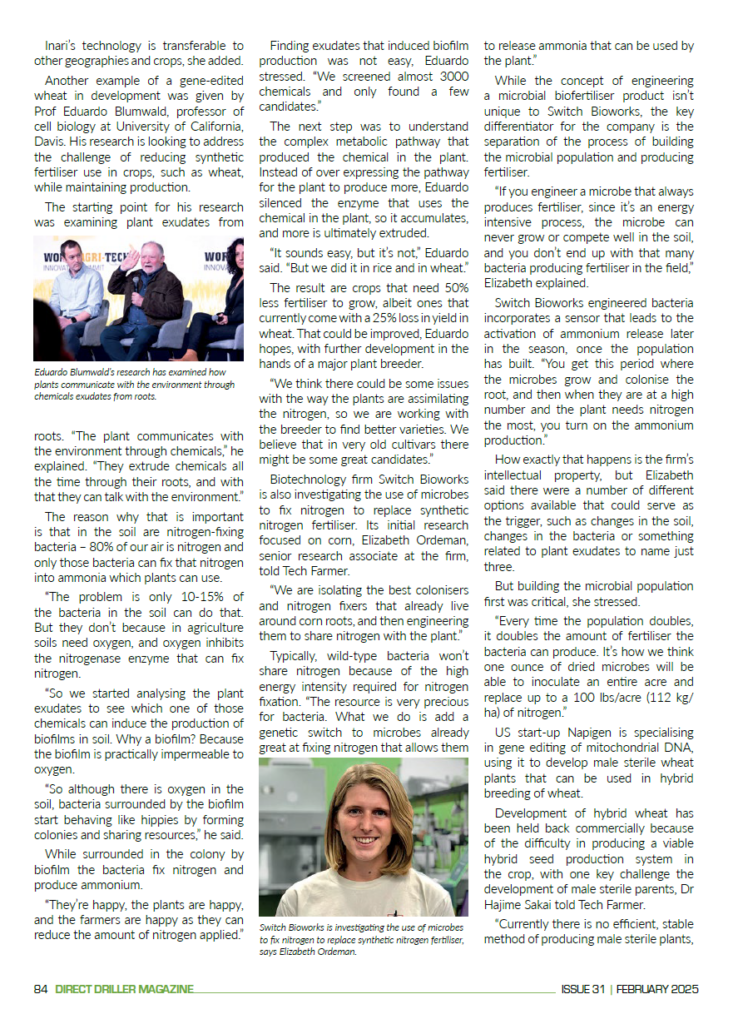
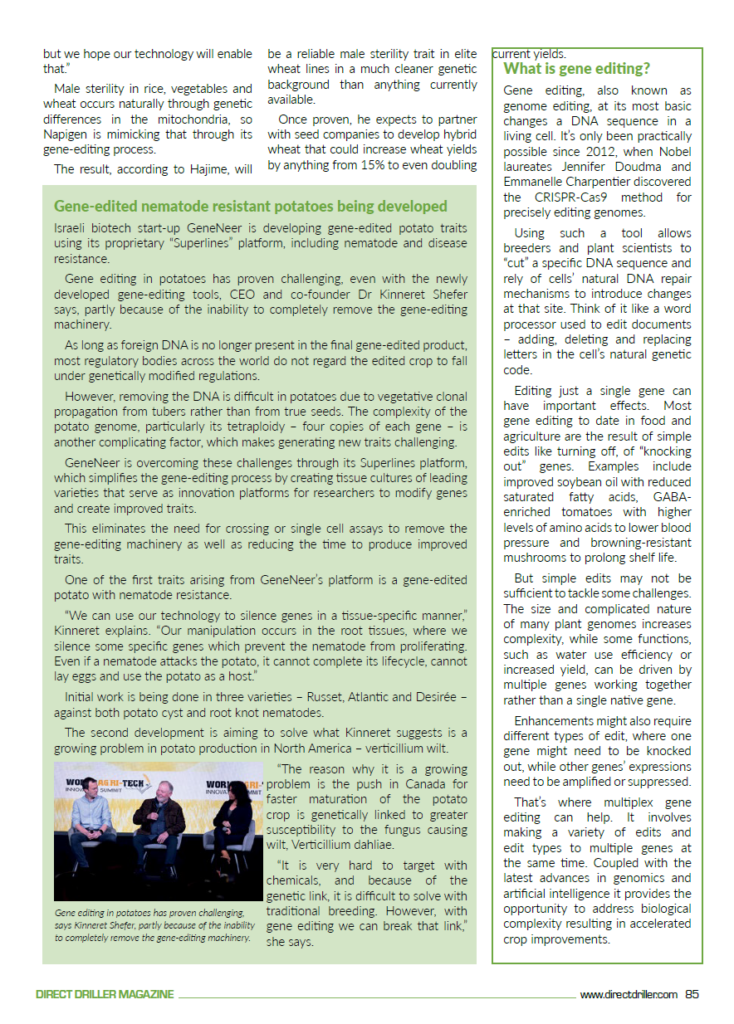