Written by Keith Diedrick, Area Agronomist at Corteva USA
Summary
• Soil testing is a useful and relatively inexpensive management tool for growers to assess crop nutrient levels in their fields.
• Proper methods and timing of soil sampling help ensure reliability of test results for making informed decisions related to soil inputs such as fertilizer and lime.
• Standard soil tests typically evaluate pH, buffer pH, organic matter, cation exchange capacity, phosphorus, potassium, calcium, magnesium and base saturation.
• Micronutrient soil testing alone is generally not a reliable tool to predict potential micronutrient deficiencies. Tissue testing, soil properties, and growing conditions together are a more complete diagnostic approach.
• Critical levels of nutrients (the point below which crop yield may decrease quickly) and crop removal rates are useful for determining the rate and frequency of fertilizer application to a field.
Growers must efficiently manage field inputs while reducing the risk of yield losses to maximize profitability season after season. Fertilizers are significant variable costs in production, and tools are available to assess their need.
Soil Testing – Selecting a Laboratory
Competent analytical laboratories use strict standardized methods in controlled environments with quality standards; all of these are important to reliable and comparable data for informed decision making in the field. A number of commercial labs exist and some land-grant colleges have labs as well. Growers may benefit from a review of considerations and tips for selecting a soil testing laboratory (Diedrick, et al. 2010).
Soil test kits and strips found at garden centers are not a replacement for a professional testing laboratory analysis due to the risk of high variability and questionable precision. A good laboratory test report is a small investment compared to the magnitude of fertilizer and lime investments by an operation. Once a laboratory is selected, a quality sample must be collected and handled correctly for an accurate analysis.
Sample Collection
Regardless if the sampling plan is random or part of a precision management system, collecting and submitting a clean and representative sample is required for reliable results. Below are some best management practices for obtaining representative samples for analysis.
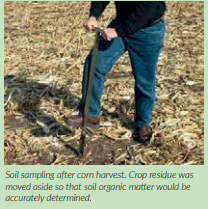
• Soil sample every 2 to 4 years for a given field; sampling every year rarely adds additional information.
• Sample when crops are not growing in the field (applies to standard soil sampling).
• Avoid fields where fertilizer, manure, or liming materials were recently applied.
• Sample fields at the same time
every year so that analyses are more comparable over time. Sampling 3 to 6 months prior to the next crop will allow enough time for any pH or nutrient adjustments. For many crops, this time is post-harvest in late autumn.
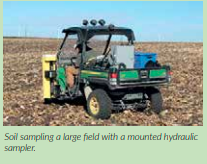
• Thoroughly clean the soil probe or instrument to remove all residual soil or debris. It does not take much of a foreign substance to significantly contaminate a sample.
• Be sure the sampling container is similarly clean to prevent any contamination. Plastic or stainless steel containers are preferable to other materials.
• Move residue, debris, and any vegetation from the soil surface at the sample site. Failing to do so will cause an incorrect (too high) organic matter measurement.
• Pull soil cores from a depth of 6 to 8 inches, or depth to plough layer where soil mixing occurs. Long-term no-till fields, perennial forages, ridge tillage, and similar systems may be sampled also at a shallower depth to note any shift in soil pH in the top 2 to 4 inches.
• Obtain 15 to 20 soil cores for an area of 20 acres or less. For larger areas, submit multiple samples for more accurate soil information, even if the field appears uniform.
• Mix cores together well for the sample. Usually, samples can be sent moist after mixing. For standard soil tests, air drying is permissible, but do not heat cores to speed drying.
• Follow the soil laboratory’s instructions for submission, using the containers recommended or supplied by the lab.
A Typical Standard Soil Test Report
Most laboratories offer a “standard soil test” which covers a set of soil chemical measurements that are important in agronomic decision making. Though some labs vary, typically this includes pH, buffer pH, cation exchange capacity (CEC), base saturation, organic matter (OM), and the macronutrients phosphorus (P), potassium (K+), calcium (Ca2+), and magnesium (Mg2+). In the Plains and western regions where salinity and sodicity are more common, conductivity, soluble salts, sodium (Na+) and chloride (Cl-) may also be reported in a local laboratory’s standard test. Nitrate (NO3-) and sulphur (S), are not usually part of standard tests, but are considered macronutrients. Nitrate tests are used in some regions for preplant nitrogen (N) management and in-season sidedress decisions, but recommendations vary from region to region.
Test Values Explained
pH is the measure of acidity of a substance. pH is expressed as a number, not a percentage or other quantity. A value of 7.0 on the pH scale is considered neutral, that is, the substance is neither acidic nor basic. Lower numbers on the pH scale denote increasing H+ ions and acidity. The acidity of the soil solution affects many physical, chemical, and biological reactions necessary for plants to survive and thrive. The optimum pH value varies by crop and region, but is generally between 6.0 and 7.0 pH (slightly acidic), though alfalfa thrives between pH 6.8 to 7.0.
Buffer pH – Once a need for adjusting pH higher is identified, the buffer pH value indicates the amount of liming material necessary to make the adjustment. Low buffer pH values indicate that more lime materials are necessary to raise pH than higher buffer pH values. “Buffering” in this case refers to the ability of the soil to “recharge” acidity, and is a function of aluminium (Al3+) minerals and H+ in the soil (and is related to CEC). If a soil is poorly buffered (sandy), less liming material are needed to raise pH a certain level than a soil that is highly buffered (clayey soil). Some soil tests may report buffer pH as lime test index, or LTI, which is merely the buffer pH value multiplied by 10.
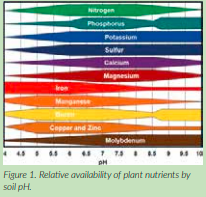
Organic Matter – Expressed as a percentage, OM is the non-mineral content of the soil sample and is usually determined by combustion. Organic matter has many functions, including water-holding capacity, nutrient cycling, and contributing to soil structure and CEC
CEC – Cation Exchange Capacity is measured in milliequivalents per 100 grams of soil. CEC is determined primarily by soil clay mineralogy and OM level in the soil. Cation exchange capacity represents a measure of electrostatic charge sites in the soil that can hold cations, or positively charged ions, like Ca2+, Mg2+, Mn2+, Zn2+, K+, H+, and Al3+.
For each “equivalent” charged site on the soil particles, a single positive charge can be held (denoted by the superscript number on these ions). In the case of K+, a K+ ion has a single positive charge, and is held by one CEC site. For a Ca2+ ion, two CEC sites are necessary to hold one Ca2+ ion, three CEC sites for Al3+, etc.
Higher CEC values in soil represent more capacity for nutrient cations to attach. For example, a high CEC soil can theoretically hold many K+ ions, but would require more K+ to be applied as fertilizer to fill the sites located on the soil particle surfaces before it is easily released to the soil solution and taken up by plants
P – Phosphorus is reported in parts per million (ppm) or pounds per acre (1 pound per acre = 2 parts per million). This is not a measurement of total P in the soil, however, it is an estimate of P that is available to plants. The testing procedure utilizes an extractant that is correlated with P uptake that might occur by plant roots. This value is reported on the soil test as “extractable P.” Not all labs use the same process; some may use the Bray-Kurtz P1, Mehlich III, Olsen, or other procedure. Reported P values will vary according to the test procedure and are not directly comparable to each other.
P is an important element in plants, as it is part of the DNA, RNA, the energy transfer molecule ATP, as well as amino acids and proteins.
K+ – Potassium is reported as ppm or lb/acre of “exchangeable K.” Like P, all of the K+ in a soil sample is not available to the plant. Most of the K is locked in mineral structures, some is available slowly from the clay edges, and other K+ ions may attach to the CEC (exchangeable) and flow easily to the soil solution. The K+ extraction solution measures K+ that can be readily moved from the CEC and into soil solution, simulating the processes that might exist with a root system in the soil
Potassium is vital in water regulation and enzyme activation in plants. Plant stomata, which are openings in the leaf used for gas exchange, open and close by movement of K+ in and out of the cells surrounding the opening. Potassium (and other nutrients) in plant stover or residue on the soil surface can cycle back to the soil with rainfall events and residue breakdown (this sometimes includes up to 80% of K in residue). If little to no rainfall occurs after harvest prior to soil sampling, the reported K+ value may be lower than expected because of this lack of cycling. Dry conditions also limit the movement of slowly available K+ to the CEC.
Ca2+ – Calcium content of the soil is reported in ppm or lb/acre, sometimes listed as “exchangeable calcium.” Calcium is a common element in a lot of mineral soils, and though they may occur in some regions, deficiencies are rare in most soil environments above pH 5.5. The easiest correction to a suspected Ca2+ deficiency is raising soil pH with a liming compound, and Ca2+ is considered sufficient at ≥200-300 ppm. Calcium is essential to plant cell wall structure, cell division, and many enzymatic processes.
Mg2+ – Magnesium levels are measured in ppm or lb/acre, sometimes listed as “exchangeable magnesium.” Magnesium is a key element in chlorophyll and many enzymes and enzyme activation. Deficiencies can occur in crops, and can be remedied with Mg-containing fertilizers or dolomitic limestone. Mg2+ is sufficient for most crops at ≥50-100 ppm.
Base Saturation – This is a description of the major cations held on the CEC on a percentage basis. Typically, Ca2+, Mg2+, K+, and H+ are listed (and sometimes Na+ and Al3+) as they are of the highest concentration. Other cations may attach, such as the metal micronutrients, but they are often measured in ppm, not a percentage (1 ppm = 0.0001%) The percentage of H+ is related to the buffer pH value discussed earlier. When H+ moves off of the CEC and into soil solution, the solution becomes more acidic. The portion of H+ on the CEC is sometimes referred to as “reserve acidity.
Base Saturation Ratios
There is a long-standing debate on the usefulness of base saturation for determining lime and fertilizer rates, particularly the Ca:Mg ratio. There is a lack of evidence that a certain ratio of bases is necessary for optimum crop performance. A large body of research shows that if nutrients are in the soil at high enough levels and pH is in the proper range, optimum yields in agronomic crops can be achieved across a wide range of Ca:Mg ratios.
There are a few ratios of note for specific crops and soils, however. The ratio of Mg:K is of importance to forage growers. If excessive K+ is taken up by the plants and Mg2+ is low, the forage may contain low Mg2+ content and cause a metabolic condition in livestock called grass tetany (hypomagnesemia). Dolomitic limestone and Mg-containing fertilizers are options, but supplying feed additives with Mg to livestock may be more economical.
When Mg2+ exceeds Ca2+, or when Na+ becomes high (>15%), this may be indicative of possible soil structural issues, such as clay dispersion or flocculation, surface sealing, and decreased water infiltration. This is most likely to occur in areas of naturally occurring Na+ and Mg2+, but these are not widespread in North America.
Soluble Salts – The soluble salt content (or conductivity) is expressed in dS/m or mmho/cm (which are equivalent). If soluble salts are too high in the soil, plants cannot take up sufficient water, wilt, and in severe cases, die. In most crops, performance and yield decline rapidly at 3 to 4 dS/m and higher, and may be affected as low as 2 dS/m.
Salt problems are a concern primarily in the Plains and western regions of North America where various salts are native to the soil in high levels. Some effluent irrigation waters and fertilizers have the potential to cause salt problems as well, most notably muriate of potash (KCl; 0-0-60) and urea (46-0-0). Use extreme caution when applying in-furrow or banding.
Micronutrient Soil Tests
Notice that standard soil tests typically do not include elements like manganese, boron, zinc, iron, and copper. At this time, it is difficult to correlate micronutrient levels in the soil with crop response. Plants require very little of these micronutrients to thrive and the exact required amount can be difficult to ascertain. The challenge for researchers is to reliably correlate a soil test level with plant uptake (like P and K). Even if this was known, the relationship to test level and crop response is similarly variable.
To further complicate micronutrient predictions, the availability of micronutrients can vary with soil physical characteristics which change during the year (moisture, aeration, microbe activity, etc.). If soil test levels of a micronutrient are high, crop responses to fertilization of that nutrient are unlikely. If a micronutrient level is marginal or low in the soil, the crop still may not respond to a micronutrient application. Additionally, not all crops have the same sensitivity to particular micronutrients. Knowing the soil, crop sensitivity, and environmental factors that may cause a nutrient deficiency is helpful to gauge risk and prepare appropriately (Butzen, 2010; Diedrick, 2010; Jeschke and Diedrick, 2010).
Choosing Fertilizer Rates
A starting point in nutrient management decisions is comparing soil test results to established critical levels for the particular crop. Critical levels are the point where yield loss potential increases quickly if soil test levels for a particular nutrient fall below that level (Figure 2).
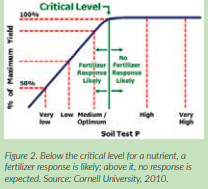
These established critical levels may vary from region to region, but recent research shows that critical values are close to the ranges in Table 1.
1 Critical level for ppm K = 75 + (2.5 x CEC) for all crops
2 Values in parentheses are lb/acre.
3 Several sources do not cite CEC specific K, and may vary. Consult your local Pioneer professional for additional local information.
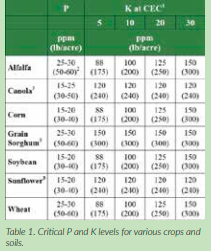
One strategy that growers may adopt is to build soil test levels just above the critical levels and apply nutrients to annual or biennial crop removal rates. This accounts for variability in fertilizer spreading as well as assuring soil test levels over critical levels for anticipated crop removal. This practice may guide appropriate fertilizer rates for rented land or when fertilizer prices are high while still reducing the risk of yield loss. Approximate crop removal rates are below; these values may vary by year and growing environment.
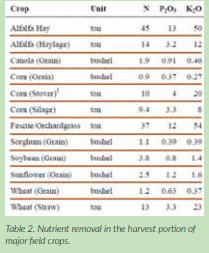
Adapted from Warnecke, et al., 2004; IPNI, 2010.
1Based on Pioneer research. Fertilizer products will have an analysis printed on the bag or bin that identifies the amount of N, P, and K contained in the product. This is widely done with three numbers arranged as N-P-K. The N value on the tag is simply a percentage of N, however, P and K are listed as P2O5 and K2O.
Crop removal and fertilization are activities that change soil test levels of nutrients. Depending on the buffering capacity (CEC) and soil mineral composition, the amount of P2O5 and K2O necessary to change soil test P and K will vary and in some cases, be difficult to predict. Research from Kentucky (Thom and Dollarhide, 2002) shows that for low levels of soil test P, more P2O5 fertilizer is required to change the levels than at high soil test P levels >100 ppm (Table 3).
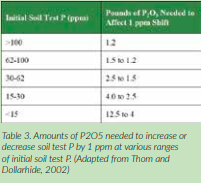
To change K+ test levels by 1 ppm, K2O additions may range from 2 to 6 pounds per acre, again, depending on existing K+ levels, soil minerals, and CEC.
Conclusions
Soil testing is an inexpensive practice to learn about the ability of soils to support crop growth. With knowledge of what each soil test value means, growers can make more informed crop input decisions to minimize risk and maximize profitability.
References and Further Reading
Butzen, S. 2010. Micronutrients for crop production. Crop Insights. Pioneer Hi-Bred Int., Inc., Johnston, IA.
Cornell Univ., 2010. Northeast Region Certified Crop Advisor study resources. Competency area 3 – soil testing and plant tissue analysis.
Diedrick, K.A. 2010. Manganese fertility in soybean production. Crop Insights. Pioneer Hi-Bred Int., Inc., Johnston, IA.
Diedrick, K.A., G. Gao, R.W. Mullen, and M.E. Watson. 2010. Choosing a soil analytical laboratory. OSU Ext. Bull. HYG-1133. Ohio State Univ., Columbus, OH. [Online
Havlin, J.L., J.D. Beaton, S.L. Tisdale, and W.L. Nelson. 2005. Soil fertility and nutrient management: an introduction to nutrient management. 7th ed. Pearson/Prentice Hall. Upper Saddle River, NJ.
International Plant Nutrition Institute. 2010. Nutrients removed by harvested portions of crop. IPNI, Norcross, GA.
Jeschke, M. and K.A. Diedrick. 2010. Sulfur fertility for crop production. Crop Insights. Pioneer Hi-Bred Int., Inc., Johnston, IA.
Kansas State Univ. 1998. Grain sorghum production guide. K-State Res. and Ext., Manhattan, KS.
Kansas State Univ. 2009. High plains sunflower production handbook. K-State Res. and Ext., Manhattan, KS.
Seelig, B.D. 2000. Salinity and sodicity in North Dakota soils. NDSU Ext. pub. EB-57. North Dakota State Univ., Fargo, ND.
Thom, W. and J. Dollarhide. 2002. Phosphorus soil test change following the addition of phosphorus fertilizer to 16 Kentucky soils. Univ. of Kentucky Coop. Ext. Agronomy Notes, 34(2). Lexington, KY.
Vitosh, M.L., J.W. Johnson, and D.B. Mengel. 1995. Tri state fertilizer recommendations for corn, soybeans, wheat and alfalfa. Ext. Bull. E-2567. Michigan State Univ., Ohio State Univ., and Purdue Univ.
Warncke, D., J. Dahl, L. Jacobs, and C. Laboski. 2004. Nutrient recommendations for field crops in Michigan. MSUE Bulletin E2904. Michigan State Univ., East Lansing.
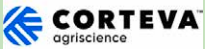